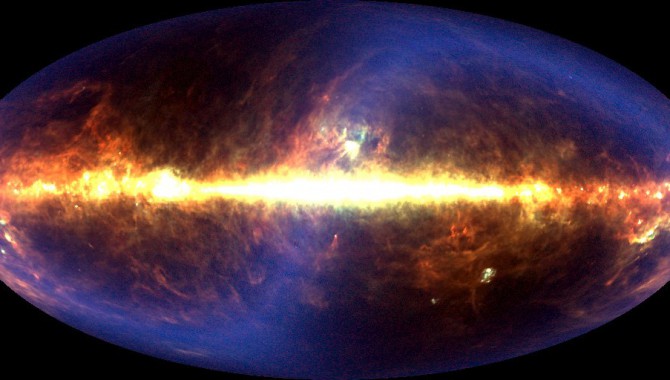
By Noel Hinners
Starting in 1963, I have witnessed in amazement the science discoveries made by the nation’s human and robotic space program. I also had a direct hand in those discoveries, first on Apollo and subsequently as NASA’s Associate Administrator for Space Science and director of Goddard Space Flight Center. As director of the Smithsonian National Air and Space Museum, I saw firsthand the impact our space and aeronautics programs have on the public, and at the University of Colorado’s Laboratory for Atmospheric and Space Physics and its aerospace engineering sciences department, I’ve witnessed their power to inspire students. NASA, especially in its forays in space science, has the capability to expand our knowledge and understanding not only of the universe around us but also of our own planet. The science done by exploring space is, at its core, an extension of physics, astronomy, geology, and chemistry—things we’ve done terrestrially for well over a hundred years. But leaving the confines of our own atmosphere has materially advanced our knowledge in those areas and offered boundless material to inspire practical applications and our imaginations.
When I started my specialty of planetary science in the early sixties, the moons and other planets were simply interesting little blobs in space. But each time we take a closer look, we find out how incredibly fascinating and complex they all are. We’ve seen evidence of geologic processes unlike those that have occurred on Earth, and what we see on Titan may mirror the most primitive beginnings of our solar system, going back to when organic chemistry was being formed. Organic chemistry, along with water, is the key to life, and the search for life remains a driver of space science. Cassini’s results at Titan have taken on incredible priority because they may give us a clue about how life started in the solar system. The recent findings of abundant past water on Mars have also invigorated the new field of astrobiology.
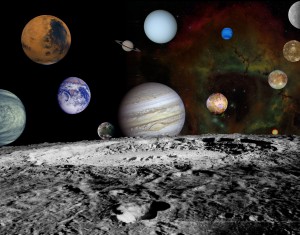
This montage of the nine planets and four large moons of Jupiter in our solar system is set against a false-color view of the Rosette Nebula. Most of the planetary images in this montage were obtained by NASA’s planetary missions, which have dramatically changed our understanding of the solar system in the past thirty years.
Image Credit: NASA, Arizona State University
The complexity and incredible diversity of geologic processes that we’re seeing in the solar system beat anything we imagined in the early sixties. Getting above our atmosphere and seeing light wavelengths that are typically obscured have broadened what we know about the evolution of the universe, galaxies, dark energy, and black holes—knowledge that helps us solve more of the mysteries about our own planet and how it fits into the grand puzzle.
Where we can explore is, as far as we know, limitless. So choosing our destinations and experiments is not an easy task. Picking one foray from among thousands—or millions—of possibilities requires coordination between NASA and the external science community, an ongoing collaboration that has been one of NASA’s major strengths.
Deciding Where to Go
Deciding which endeavors to pursue requires first asking, “Is it good science?” The proposals NASA receives come from scientists who make a career out of thinking about what’s exciting in space and how to find out more, so they can answer that question well. But there is always more science to do than can be accommodated.
NASA, like Gaul, is divided into three major parts: human space flight, aeronautics, and space and earth science. I headed up the space science part of NASA as associate administrator from 1974 to 1979, and my job was both to manage the programs we had taken on from a Headquarters perspective and to initiate new programs. Each endeavor requires being both a proponent and a manager. The Agency works closely with the National Academies’ Space Studies Board to help decide what priorities should be, asking, “Is it forefront science? Is it potentially a breakthrough? Does it add materially to our store of knowledge?”
Science prioritization was and is an important job at Headquarters. It was easier to do for small missions, because the big ones, like Galileo and Hubble, required so much of the budget it made them tougher to get approved. A big part of my job was selling the big science programs that had major science payoffs while keeping the little ones that also contributed invaluable science going. COBE was one of those small programs, and it is NASA’s only Nobel Prize–winning mission.
Beyond Science and Engineering
NASA’s collaboration with the external science community doesn’t end once a destination has been chosen. Once we have a destination, we have to figure out how to get there and achieve the science experiment. After all, the experiments—and a means of getting them into space—still need to be created. Communication between scientists and engineers is essential for a project to succeed. These two communities speak different languages, however, and it’s not always easy for them to understand one another. By having a quality in-house science community, NASA has been able to forge a better link between its engineers and the academic science community.
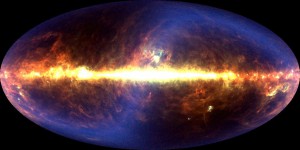
Small explorer missions like the Cosmic Background Explorer (COBE) contribute to space science as much as the larger missions, like Hubble. COBE won a Nobel Prize and helped prove the big bang theory. The above image, in representative colors, is a projection of the entire infrared sky created from years of observations by COBE.
Photo Credit: NASA
Excellent engineering and science are the sine qua non of mission success, and NASA has the skills to solve technical issues, but these alone are insufficient. Failure reviews from Challenger, Columbia, and the Mars ’98 missions consistently point out how a lack of genuine communication is often a major contributor to problems. Communication is in and of itself an art form that must be cultivated. We must ask, are we talking to one another in terms we understand? Are we listening with intent to hear? Have we properly aligned roles and responsibilities so we’re not getting in each other’s way? Are we working in a cooperative mode where everybody is providing the skills and capabilities that bring a project together? Communicating, understanding one another, and defining roles and responsibilities are essential necessities for project success. NASA clearly recognizes this and actively supports team development to hone communication skills and should continue this practice.
Once the right team is in place and communicating well, many missions face the budget hurdle. Stringent budgets have been with NASA continually, even during the latter part of Apollo. This presents the challenge of figuring out how to do missions more economically, do them more quickly, get more for the investment dollars, and push the boundaries without falling into the downside of faster, better, cheaper. I was involved in the infamous faster-better-cheaper era, which resulted in the Mars mission failures of the late nineties. But they didn’t fail because faster, better, cheaper is inherently a bad concept. When you take the practice to extremes and try to do things for too little money, though, you get into trouble. We should have been smart enough not to push ourselves to attempt things that didn’t make good engineering sense. It was a painful lesson and one we need to transmit to those who are coming into the business today.
From Discovery to Further Exploration
Mars has long been high on the list of places to go. It has had a special place in science programs and in dreams about human exploration. Much of this fascination was driven by both the fantasy and actual potential for finding life. The early Mariner flybys and orbiters set the stage. And then, in 1976, Viking landed with a prime goal of searching for evidence of life on Mars. While obtaining a lot of geologic and atmospheric information that significantly improved our understanding of the Mars environment, it detected no evidence of life. This is not because there never was or never might have been primitive life, but because all the evidence we’ve gathered so far shows that Mars has a very chemically active, oxidizing surface, which destroys organic molecules. This active surface remains today, even at the Phoenix site. It is one of the most intriguing and damning aspects of Mars. The surface is so oxidizing, I wonder if humans can cope with it. Can we devise life support systems for astronauts that will enable them to be safe? This is a major question as regards future human exploration of Mars.
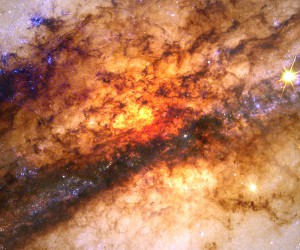
Balancing larger missions with smaller ones is an ongoing process at NASA. Larger missions require more money and can be tough to get approved, but the dividends are worth it, as shown by this image of the Centaurus A galaxy from the Hubble Space Telescope.
Photo Credit: NASA, E.J. Schreier (STScI)
A Mars sample return (MSR) mission has the potential to significantly advance our understanding of all aspects of Mars, especially the life question. Getting a sample back will enable more precise and detailed science in a terrestrial laboratory than we can possibly do remotely today. While remote-sensing experiments improve, they’re always ten to fifteen years behind what we can do in a terrestrial lab; it simply takes time for an instrument to evolve from lab use to a flight experiment. With that detailed science, we can better understand the oxidizing surface awaiting us on Mars, an issue that must be resolved before we can be serious about sending humans. MSR is also an opportunity to begin structuring a round-trip mission to the red planet. In that sense, the sample return mission is a prototype for potential human exploration.
To achieve MSR, of course we’ll need the obvious: the right leaders, science team, and budget. It will be an expensive mission, on the magnitude of the big astronomy missions: what Hubble has been and the James Webb Space Telescope will be. One way to help tackle the budget challenge is to use international cooperation. The European Space Agency and others are very interested in MSR, and NASA is working with potential partners to see what kind of cooperative MSR mission would make sense.
Looking into the Future
No one can accurately predict NASA’s next fifty years. Look back at the fifties and sixties and see what people were predicting then. Wernher von Braun’s Collier articles envisaged monster orbiting space stations and humans on Mars. Those things haven’t happened. On the other hand, we totally underestimated the direction things would take and the advances in technology that are, in large part, unpredictable beyond a decade or so. Our robotic capability has improved unbelievably over the past fifty years. Even forty years ago, few conceived of doing some of the things we do today robotically.
I did a thought experiment and concluded that today we could do robotically most of what Apollo did. As proof of this concept, note that the Spirit and Opportunity rovers on Mars exceed in capability the geologic exploration capability of Apollo in many ways. There’s no reason at all that our progress in robotics will do anything but continue to evolve, which brings up a major challenge for NASA: how to balance human exploration with things you can do robotically at one-tenth or one-hundredth of the cost. And while robotic approaches may be more cost-effective, humans have special capabilities that are incredibly useful in exploration. The Hubble repair and refurbishment activities are a prominent example. We need to do a much better job of understanding how to integrate NASA’s human and robotic worlds. Finding the right mix of humans and robotics is a major challenge that the agency needs to face.
In the future as in the past, NASA will have to choose how best to use scarce resources. Good communication and strong engineering and science skills within NASA, industry, and academia will all remain important. Although it’s not possible to predict exactly where we’ll be decades from now, I think it’s safe to say that NASA will continue to make astounding, unexpected, and valuable scientific discoveries.