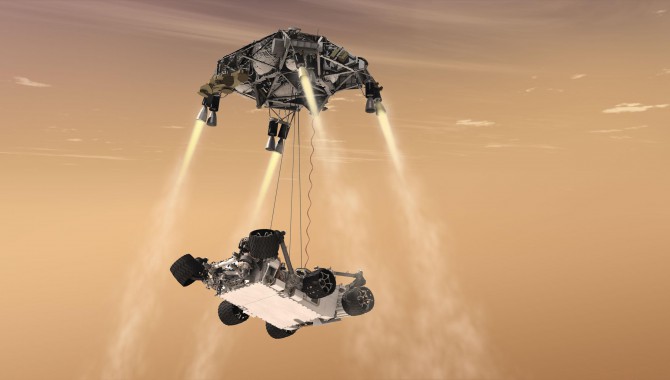
By Don Cohen
For all these missions, entering and descending through the Martian atmosphere and putting an undamaged lander on the surface (the mission phase known as EDL, for entry, descent, and landing) has been technically demanding.
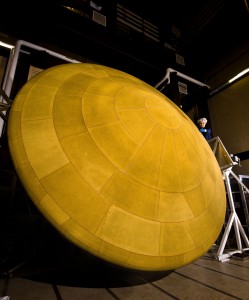
The finished heat shield for Mars Science Laboratory, with a diameter of 4.5 meters, is the largest ever built for descending through the atmosphere of any planet.
Photo Credit: NASA/JPL-Caltech/Lockheed Martin
It is much harder than landing on the moon—in part because of the planets greater mass and gravitational pull, but especially because Mars has an atmosphere that heats and exerts shear forces on objects moving rapidly through it, as well as strong winds that can blow a spacecraft off course. And the relative thinness of Mars’s atmosphere (it is less than 1 percent as dense as Earth’s and as rarified at the surface as our atmosphere is at 100,000 feet) means it is not substantial enough to slow and land a sizeable spacecraft with frictional heating and parachute drag alone—the method used for Apollo and Soyuz space capsules returning to Earth.
Past Mars missions have used a variety of techniques to solve the problem. The Pathfinder and Mars Exploration Rover (MER) missions used parachutes and retrorockets to slow the spacecraft and airbags to cushion the landers and rovers when they dropped to the surface. But the Mars Science Lab (MSL) team quickly determined that airbags would not be a viable solution for something as big as Curiosity. An airbag system designed to accommodate the size and mass of Curiosity would be very large and heavy and significantly different from the Pathfinder and MER designs. In addition, egress from the top of the deflated airbags and lander platform is a complex and tricky maneuver; it would be even more complex with an airbag design large enough for Curiosity.
Along with the huge difficulties presented by the physics of landing a large spacecraft on Mars, there are the challenges and pitfalls inherent in any ambitious mission—the mistakes to be avoided, the risks to be anticipated and eliminated or minimized. As Miguel San Martin, who designed the guidance and navigational controls for the mission, says, “There are the problems Mars creates for you, and the problems you create for yourself.” A lot of learning, experience, design, testing, and review has gone into solving or avoiding both kinds of problems.
The novel solution the MSL team has developed is what they refer to as a “sky crane.” After reducing its speed through a combination of atmospheric friction, parachute, and retrorockets, a descent stage with Curiosity hanging from it in a bridle of nylon tethers will use its thrusters to essentially hover as it lowers the rover to the surface—”a way of landing without landing,” in the words of Steven Sell, who is responsible for verification of the EDL system. After touchdown, the bridle will be cut and a 6-second burn will ensure the descent stage crashes some 400 meters away.
Learning from the Past
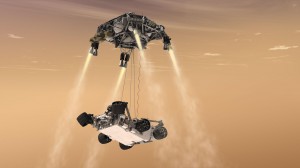
This artist’s concept shows the sky crane maneuver during the descent of the Curiosity rover to the Martian surface.
Image Credit: NASA/JPL-Caltech
After the failures of the Mars Surveyor and Mars Climate Orbiter missions in the late 1990s, it became clear that a Mars sample-return mission projected to launch in 2003 would be canceled. Knowing the mission would not go forward but still funded for a time, the sample-return team decided to devote their efforts to going back to first principles and think about all the ways to design a lander and put it safely on the surface. One of the questions they explored was whether it was possible to get velocity control so good that you could land a wheeled rover directly on the surface, rather than cocooned in a lander. (At the time and for a couple of years afterward, the answer was no.) Rob Manning, now the chief engineer for Curiosity and, years earlier, the chief engineer for Mars Pathfinder, wrote to the chief engineer of the sample-return team, asking, “Have you thought about helicopter mode?”—what was also known at the time as “rover on a rope.” This was 1999, and they passed on the idea for fear of the two-body pendulum dynamics inherent in the architecture. With two bodies connected by tethers, there was concern over the potentially chaotic dynamics of the swinging pendulum motion that might result. Ultimately, this was a controls problem.
The concerns of the sample-return team represented an essential hurdle for the creation of Curiosity and the sky crane. Perhaps the key ingredient to getting past that hurdle was the experience San Martin had taken from MER development. MER had taught San Martin that he could effectively steer the pendulum and control the two-body dynamics, the key requirement to attempt what would be called the sky crane maneuver. The MSL EDL team even brought in a helicopter pilot from Sikorksy to apply his experience to plans for EDL. As Manning says, “Had they started from scratch, they never could have achieved this.”
The sources of earlier experience they drew on included the Viking mission, which reached Mars more than three decades earlier. The engines on Curiositys descent stage are an upgraded “reinvention” of Viking’s throttleable engines, which the MSL team developed by studying available Viking documentation (which was not as comprehensive as theyd hoped), talking with Viking people, and reverse engineering still existing Viking-era engines. According to one team member, they “scrounged up” all the Viking data they could, a search that included locating an informative film of a Viking parachute test in the attic of a NASA retiree.
Parachute experience on Pathfinder and MER has also contributed to the MSL design. Getting as much information as possible about the behavior of large supersonic parachutes has been essential, especially since the MSL chute will be larger and will deploy at a higher speed than similar systems on past missions.
Another issue is the danger of aerodynamic interactions between the reaction-control system thrusters and the atmosphere, which, in the worst cases, can result in control reversal. As the plumes of retrorockets flow over the backshell of the descent stage, they can generate forces—like the lift created by air flowing over an airplane wing—that create undesired motion contrary to the intended one. The MSL design had to avoid that possibility. The team again went back to study their history, looking at Mercury, Gemini, and Apollo data; they even took a trip to the Virginia Air and Space museum to look at one of the few Apollo capsules. The EDL team deployed a series of cutting-edge computational fluid dynamics analyses and scaled tests to select thruster positions and orientations and verify that the resulting aerodynamic interactions were acceptable. The MSL team even passed on their finding to the Phoenix team, a tip that led the Phoenix staff to choose to turn off their entry reaction-control system for fear it might generate control reversals.
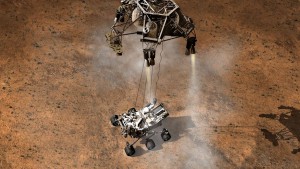
An artist depicts the moment that NASA’s Curiosity rover touches down onto the Martian surface.
Image Credit: NASA/JPL-Caltech
MSL’s large heat shield was another challenge. The team determined that the shield material they originally planned to use would not survive the shear forces created as the spacecraft entered the Martian atmosphere at high speed. The team wanted to use SLA-561V, which had worked on all the past Mars missions from Viking on. They baselined SLA and started testing it. The old standard seemed to be working until some of the final tests in June 2007, when things went very wrong.
“I was presenting the state of our EDL development at the project CDR [critical design review]. We thought everything was going well, including the TPS [thermal-protection system] testing, which was almost complete,” said Adam Steltzner, who led the EDL development for MSL, “when all of a sudden my cell phone starts vibrating in my pocket with news of a TPS testing failure. The SLA had just dissolved in testing—complete failure!”
They were short on time for the 2009 launch and needed to solve this problem quickly. The team conducted a rapid search of possible replacement materials in a short-turnaround, make-or-break trade. “We really did not have much time to make the 2009 launch date,” said Steltzner. They ended up with a heat shield made of PICA (phenolic impregnated carbon ablator). A lightweight PICA heat shield had been used on the Stardust sample-return mission, and SpaceX uses a PICA shield on its Dragon capsule. NASA’s Crew Exploration Vehicle (CEV) team studied the material extensively. Although they eventually decided not to use PICA, their research was a tremendous boon to the MSL team.
That CEV work is one of many examples of research and experience elsewhere in NASA contributing essential knowledge to Curiosity. As Manning says, “The NASA community as a whole should be proud of MSL. Only Apollo and shuttle have brought NASA together to this extent.”
There were challenges, but, says San Martin, “No developmental shoe dropped during design and development”—that is, no major weaknesses in the concept were uncovered. Early, relatively small surprises meant small tweaks, but, he adds, “The final product looked like the early sketches.” That is a testament to a well-conceived design, a point of pride for San Martin, arguably the most important contributor to the sky crane’s architecture.
Although the team was able to get the EDL systems for Curiosity ready in time for the 2009 launch, the rover’s wheel-drive actuators and avionics hardware could not make the launch date. The project ultimately slipped to the next favorable date for launch to Mars, in 2011.
The Skeptics Test
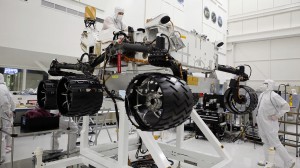
Engineers working in a clean room at the Jet Propulsion Laboratory installed six new wheels on the Curiosity rover.
Photo Credit: NASA/JPL-Caltech
Convincing people outside the team that the sky crane was the right solution for Curiosity took some doing, maybe because it is hard for people to give up their long-standing idea of what the “right” landing architecture is—that is, setting down on legs with the engines below the lander. That describes the lunar landings, of course, as well as the classic landing procedure in hundreds or thousands of science fiction stories and films.
The MSL team rounded up skeptics—veterans of Viking, Apollo, and the Delta Clipper reusable launch vehicle program, among others—to test themselves, to “make sure we’re not all drinking the Kool-Aid together.” As expected, the assembled skeptics picked at details of the plan, saying, “I don’t trust this; I don’t trust that.” A main concern was those two-body pendulum dynamics that had stopped the use of the architecture the first time back in 1999.
A year later, when they brought the group together again, the team had solid answers to all those concerns. The process was repeated—more doubts expressed; those doubts set to rest a year later—until the skeptics were convinced and the team was confident that the sky crane would work.
No Mars mission is certain, obviously, but the team believes the likelihood of a successful EDL is very high. “We have margin all over the place,” says system engineer Al Chen. Other team members agree that the risks are lower and the margin for error greater than on past Mars landings. In part, that is the result of having analysis and simulation tools that are an order of magnitude better than what was available for earlier missions. More computer power means better virtual testing; they have carried out more than 2,000,000 Monte Carlo landing simulations—randomly generated possible sequences of events played out on computers.
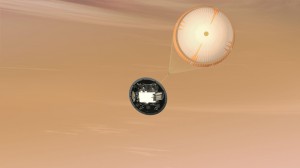
The Mars Science Laboratory mission will use the largest parachute ever built to fly on a planetary mission.
Image Credit: NASA/JPL-Caltech
Partly, though, the sky crane landing architecture is clearly more robust than other options. For instance, landing on Curiosity’s six wheels is inherently more stable than landing on legs. With a landing on legs, accurate touchdown detection is critical because a retrorocket burn of even a few milliseconds too long threatens to tip over the lander. A wheeled rover like Curiosity has much more leeway—a full 1.5 seconds for cutting the bridle connecting the rover and the descent stage.
Planning for the Future
As ambitious as it is, the Mars Science Laboratory mission is only one step in the ongoing history of planetary exploration. Vividly aware of how important their own learning from past missions has been, the MSL team is taking care to store documents detailing their work in an EDL repository that will be available to future project teams.
Equally or more important, they say, at least in the near term, is that “people will spread out.” Just as veterans of Pathfinder and MER brought their hard-earned expertise to MSL, members of the MSL team will go on to join other project teams and apply the knowledge they gained from their Curiosity work to the next generation of entry, descent, and landing challenges.
Related Links
More Articles by Don Cohen
- In This Issue (ASK 46)
- Interview with Lisa May (ASK 46)
- In This Issue (ASK 45)
- In This Issue (ASK 44)
- In This Issue (ASK 43)
- View More Articles