NASA Research Engineer Ryan McClelland discusses digital engineering technologies that are transforming classical engineering tasks and processes.
The evolved structures process, which encompasses generative design and digital manufacturing, uses computers and artificial intelligence (AI) to design and make parts, and is especially well-suited for spaceflight structures. Generative design and digital manufacturing use AI and robots to build lightweight structures, which could significantly reduce the mass of spaceflight hardware as well as development time. Generative design represents a paradigm shift in the design process, allowing engineers to define design objectives and requirements while AI generates optimized designs to meet the parameters. Digital manufacturing enables complex, lightweight designs to be robotically manufactured from 3D models.
In this episode of Small Steps, Giant Leaps, you’ll learn about:
- Differences between the evolved structures process and traditional engineering design processes
- Infusion of the evolved structures process into NASA projects
- Potential impact of these digital engineering technologies
Related Resources
GSFC Engineering Colloquium: Generative Design and Digital Manufacturing (NASA Internal)
Mars Sample Return Mission: Capture, Containment, and Return System
Optimized Space Telescope Structure: Penn State Capstone Video
APPEL Courses:
Space Mission Operations (APPEL-vSMO)
Model Based Systems Engineering Design and Analysis (APPEL-vMBSE3)
Change Management (APPEL – vTCM)
Creativity and Innovation (APPEL-vC&I)
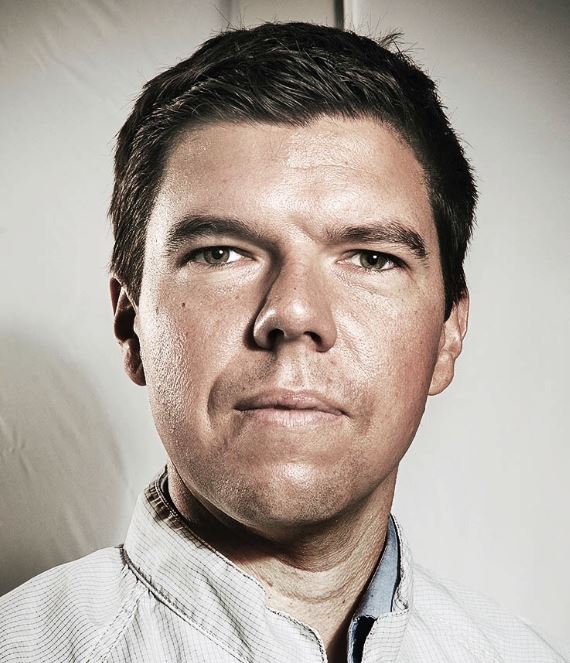
Ryan McClelland
Credit: NASA
Ryan McClelland is a Research Engineer in NASA Goddard Space Flight Center’s Instrument Systems and Technology Division focused on developing and applying digital engineering technologies. McClelland is also the lead engineer on the Next Generation X-Ray Optics (NGXO) project. His previous technology development experience includes work on aluminum foam core optical systems and non-linear effects of clearances in kinematic mechanisms. McClelland has worked on flight missions, with designs currently on orbit aboard the Hubble Space Telescope and International Space Station. He recently served as the Roman Space Telescope Instrument Carrier Manager. McClelland has authored or co-authored 60 papers on opto-mechanical systems, and his work on generative design was featured in Digital Engineering 24/7 magazine. He received a bachelor’s in mechanical engineering from the University of Maryland.
Transcript
Ryan McClelland: Those humans, maybe they do an iteration every week or two between them, if things are going well. The AI will do something on the order of an iteration a minute. So, you get a lot more iteration cycles, and because of the more iteration cycles, you just get more optimal designs much, much faster using this evolved structures process.
It’s much faster to come up with the structure, about 10 times faster, and then the structures tend to perform much better. So, they’re somewhere on the order of three times better in performance.
Almost all of our cost is non-recurring engineering, right? So, there’s only one Hubble. There’s only one Webb. So, I think these technologies are really uniquely suited to the work that we do at NASA.
Deana Nunley (Host): Welcome to Small Steps, Giant Leaps, a NASA APPEL Knowledge Services podcast where we tap into project experiences to share best practices, lessons learned and novel ideas. I’m Deana Nunley.
Digital engineering technologies are transforming classical engineering tasks such as design, analysis, and fabrication for spaceflight structures.
Ryan McClelland, a Research Engineer in the Instrument Systems and Technology Division at NASA’s Goddard Space Flight Center, joins us to discuss digital engineering technology, and specifically the evolved structures process.
Ryan, thank you for being our guest on the podcast.
McClelland: Yeah, I’m excited to talk to you about this evolved structures technology.
Host: How would you define or describe the evolved structures process?
McClelland: So, at a really basic level, it’s a new way of using computers to come up with designs. So in the past, people would just sort of invent things based on things they’ve worked on before or things they’ve seen, and the evolved structures process is a very prescribed way of using AI to come up with a design, really for anything, but especially for spaceflight structures.
So, you start with the requirements for your structure, what it has to hold, what kind of load it has to see, and then you encode those into a piece of software called generative design, and then an AI comes up with a bunch of different options for what that design could look like, and actually evolves an optimal structure. And as it’s evolving an optimal structure, it takes into account how it’s going to be fabricated. So, it makes sure that if you’re going to be machining it, it can be machined with the tools that you have on hand. And then after you get that design, created by generative design, you throw that over to something called digital manufacturing, which is just a manufacturing process that really directly takes that CAD model from generative design and fabricates it. So it really is a way of going right from what a structure needs to do, what its requirements are, to being able to build that structure physically very quickly.
Host: What are the benefits of these technologies?
McClelland: So, there’s really two things, speed and performance. So it’s much faster to come up with the structure, about 10 times faster, and then the structures tend to perform much better, so they’re somewhere on the order of three times better in performance. And when I mean performance, I mean really the stiffness to weight ratio, so they’re very stiff and very light, and they’re also quite a bit stronger than human-designed structures.
Host: Why did you decide to pursue this work?
McClelland: So yeah, over the pandemic, I was at home and had a lot of time to think about things, and also a lot of time to read and watch science fiction. And I’m really sort of a big picture thinker, and I realize an exciting future for me is where we’re a spacefaring civilization, where there are more people living off the Earth than on it. But as a mechanical engineer at NASA, I know that it’s very expensive to build things that go into space. And if you take the idea of having lots of people living in space, and then you look at something like the space station, the space station holds six or seven people, but it’s $100 billion, so you can’t have a lot of people living in space at that scale. So I really think AI has the potential to drastically lower the cost of developing these complex systems, because it’s really great at these sort of things.
And being a mechanical engineer, I got thinking about how AI can be used in mechanical engineering to lower the cost of making these cool structures in space and helping us be spacefaring. And so that is kind of the original inspiration for it, and that’s how I found this generative design idea, and even some existing products. And then I just started fooling around with it, and then eventually through Goddard’s technology program, got a little 100-hour seed funding to see if there’s any real promise behind the technology, and then rolled that into some IRAD funding, and then from there it’s just been growing and growing.
Host: That’s so fascinating. How can AI be applied to development of spaceflight hardware?
McClelland: I think there are lots of opportunities for AI to be applied, and really any time you can come up with a good set of constraints and also some kind of good objective function, like for instance, minimize mass, or maximize stiffness, or minimize stress, you can apply AI, and that’s just in the mechanical field, but I think really all across the disciplines, it’s going to have an impact. And in the mechanical field, it really is quite amazing to see the AI come up with designs that you would’ve never come up with, and one of the really fun things about this is it comes up with designs that you would’ve never come up with, but once you see them, they make sense. You’re like, ‘Oh, this design, I understand why the AI did this, and I see how it works.’
Host: How is the evolved structures, or generative design, process different than current engineering design processes?
McClelland: Well, the way that things are designed now, it hasn’t changed in quite a long time. Computer-aided design, or CAD systems, 3D modeling, have been a pretty big change since people were on the drawing board, but there hasn’t really been a big change since then. And usually the way it works is the mechanical engineer is given a set of requirements, and sometimes they’re pretty informal, that you need a bracket or a mount that holds a detector, and it has to survive this kind of load and it has to have these kind of interfaces. So, a designer will basically just kind of imagine what that might look like, and a lot of times they’ll either start with something they’ve done before, or they’ll start with thinking about just flat plates and flat planes, because it’s kind of easy to think that way and it’s easy to design that way.
So, the designer comes up with some idea and then models it up in CAD, and then we’re pretty stove-piped at Goddard, or at NASA in general, so the designer will usually sort of throw it over to a different organization to have the stress analyst look at it to see what the modes are and what the stress is in the part. So, the stress analyst will look at it and they may change some sizes of things, but they won’t change the overall layout, because usually the stress analysts don’t do design. And there’ll be a little bit of back and forth between the analysts and the designer, but not a lot.
And then from there, usually the designer or someone else will make a drawing, and that drawing will go to the machinist or some kind of fabrication outlet. And then sometimes the machinists, well, the machinist will always look at the design. Sometimes they’ll give feedback, sometimes they won’t give feedback, they’ll just grumble about how hard it is to make, and just go off and make it anyways. So it really kicks around between say three or four different people in different organizations, and it sort of evolves between those people. So to the extent that those people are giving each other feedback, it improves, but those feedback loops are really, really slow because people go on vacations, people take training. So, between design, analysis, and design for manufacturing, checking if it can be manufacturable, that’s how the design gets made, and then after it’s made, it gets tested and verified before launch, of course.
And really what the evolved structures process does is take that back and forth that goes on between a few different people, and can take months or years depending on the project and how devoted the people are, whether they’re working on other things, and it collapses that down to something that’s all done by the computer.
So, the user enters the requirements into the system, and then the AI comes up with the design, and then tests the design by finite element analysis to make sure it works, to verify the requirements, and then it also does a fabrication simulation to make sure it can be fabricated. And whereas those humans, maybe they do an iteration every week or two between them, if things are going well. The AI will do something on the order of an iteration a minute. So you get a lot more iteration cycles, and because of the more iteration cycles, you just get more optimal designs much, much faster using this evolved structures process.
Host: And then how does that process work with NASA requirements and standards?
McClelland: Yeah, that’s something really interesting that I didn’t realize at first, but is actually a big advantage, is you can directly encode NASA’s requirements and standards into generative design or evolved structures in a way that’s very deterministic. So, a lot of times, if you have a junior engineer on a project, they might not know what all the standards are, but with the evolved structures process, the standards that go into generative design are pretty well laid out, so things like we have NASA Standard 5020 for fasteners. You’re supposed to have a 1.5 D edge distance for a hole. And then in generative design, when you make the preserve, which is, say, the part of the bolted interface that needs to be in the design, you can put that in the CAD model. And other things like, you have radii that you want to keep the part from being under too much stress, and you can specify those radii right in there.
And then also things like the loads, like what are the loads? In the early design we have this thing called the mass acceleration curve, or the MAC, and part of the evolved structures process is showing the user how to take loads from the MAC and then encode them into the generative design so that you can get the design that you want. So I think it’s a much more sort of prescribed way for an engineer to come up with a design that meets all the requirements much more quickly, and it’s especially great if you have less experienced people, because it’s all kind of spelled out in the process.
Host: What’s the risk level associated with these technologies compared with human design and traditional techniques?
McClelland: So, it’s interesting. When I first started pursuing this technology, I was enthusiastic about it, but of course, a little bit skeptical myself, because that’s what you have to maintain. And then as I went through it and I started getting these really unusual-looking, almost bone-like organic structures that generative design comes up with, people reacted to them. Some people were really enthusiastic and thought that they were awesome, and some people kind of thought that they were weird and scary looking. And that’s where people see them, and they just immediately see risk. And then as I talked to other folks in the field and compared more with human designs, I think they actually are less risky than human designs, for a few reasons.
One of those reasons is they’re just much stronger. So the way that the algorithm works to evolve the optimal structure, it tends to smooth out the stress over the whole part, so you don’t have any stress peaking. So in the applications where I’ve compared human and AI designs, the AI designs are something like three, even up to 10 times lower stress than the human designs. So when you have lower stress, that just makes everything safer.
And another really interesting thing about thinking about risk is the evolved structures process is a very deterministic way of coming up with a design, so you know what’s going on in the background, how it’s coming up with the design. And it’s interesting to think about when a human comes up with a design, what’s going on in their mind? And it really varies a lot from human to human, and even day to day. So if you were to give 10 engineers the same set of requirements for a design, you would come up with 10 different designs, and they’d vary a lot in quality between, based on how junior or senior the person was, and how much coffee they drank that day. But with generative design, it’s deterministic, and I think it can lower the risk in that way too.
And one of the failure modes that I’ve seen a lot throughout my career is that sort of back and forth that I was talking about between the designer, the analysts, and manufacturing, that’s kind of like a game of telephone, and as anybody who’s played the game of telephone knows, things get dropped at interfaces. So sometimes between the designer and the analyst, there’s some subtlety in the model that’s missed, or between the designer and the manufacturing, there’s something that takes place in the manufacturing the designer doesn’t know about. So generative design and the evolved structures process helps eliminate that, because it eliminates those interfaces. So you’re not going to get a disconnect between the modeling and the FEA, or the fabrication simulation and the FEA and the modeling, because they’re all being done simultaneously by the same tool.
And I think there’s one more thing that’s really important and that’s really, I’ve had a lot in my mind, is that being able to come up with an optimal structure really quickly allows you to run what’s called hardware-rich. So a lot of times in the NASA world, we’ll design things for literally years before we build anything to test. So the evolved structures process, if you can come up with an optimal structure in a couple days and then manufacture it in a week or two, and then you can test it, you can run hardware-rich and basically do more testing and find problems quicker.
So, another area that I’ve seen things sort of go wrong in the aerospace field is most of the problems come up when you actually try to make something, and it can be because it’s harder to make than you thought, or when you get it made, there was something that you missed. So being able to iterate on the actual hardware, not just in the design, but the physical parts, I think is a really powerful aspect of evolved structures.
Host: Is this ready for infusion into NASA projects and proposals?
McClelland: So yeah, especially for a certain class of structures. So mostly I’ve used it on sort of what I would call small to mid-size metallic structures, so things like brackets and mounts, and structures that basically hold detectors or any variety of things, which really comprise a lot of especially instruments. So for those kind of structures, it’s basically ready. It’s being used now on the drone that’s going to be flying on Titan, the Dragonfly mission. It’s being used on Mars CCRS, the Capture, Containment, Return System. So yeah, it’s definitely ready, and I look forward to being able to fly some things in space soon. And in fact, there are other groups that have flown similar things in space already.
So also, for proposals, that’s a good time to sort of start using it. Really, the earlier you can get in the process, the more powerful it’s going to be. So especially for proposals, it’s great. It can help lower the mass of your system, which the cost models that are used today, mass is cost, basically.
Host: Ryan, what are you seeing as barriers to adoption of the evolved structures process?
McClelland: Yeah, it’s really interesting. A lot of people are getting excited about this work, and I see a lot of interest from project managers and from scientists, and then I also see a lot of interest from junior engineers, folks that are really interested in trying new things. But when it comes to sort of like more of the mid-level, a lot of times, folks that are really responsible for delivering the systems, a lot of times, there’s just sort of the fear of the unknown, of trying something that’s untested. And as I mentioned previously, they look like alien bones. They look weird.
And a lot of people have the sort of misconception that it has to be additively manufactured. So it doesn’t have to be 3D-printed or additively manufactured, and that technology does have some additional risk in it, because you can have flaws in the manufacturing that you might not know about without a lot of rigorous validation. So people see these crazy-looking organic structures and think that they can never be CNC machined out of our standard materials with normal CNC machining processes, and it turns out that CNC machining has actually come way further than people think, and you can make absolutely almost anything you can imagine with five-axis CNC machining these days.
So, I think that’s one of the barriers, just that they look strange, and folks think that they would be difficult to machine. And I think just the structures field often tends to be conservative, because we never want our part to be the part that breaks. Our part, a lot of times, is supposed to be the simple and reliable part, not the new technology. A lot of times, the new technologies are reserved for the detectors and things like that, at Goddard.
Host: For anyone who may be unfamiliar with machining techniques, could you tell us more about that?
McClelland: Sure. So when most engineers think of machining of spaceflight parts, they think of what’s called, they think of milling, basically. So milling is you’ve got a turning spindle and it just removes material, and the way people think of milling, the old school mill has a couple handles on it that you turn, and it translates this milling bit around, and that’s how you move material. So especially, actually, it tends to be the engineers that know the most about milling and maybe even have one in their home that tend to have the most misconceptions about what it is these days.
So, a CNC mill — computer numerically controlled milling — and five-axis, you can really take that milling bit and you can move it in almost any direction, and they’re really, really magical to see if you look up five-axis CNC on YouTube or something. So that CNC technology has really come a long way, and it’s not as much human intervention as people might think. So there’s software called CAM or computer-aided manufacturing, where you upload, you import that CAD model from generative design into this CAM package, and then it figures out the toolpath, and depending on the sophistication of the CAM software, may require more or less human input.
And then additive manufacturing is a layer-by-layer technique. For metals, it’s usually something like laser powder bed fusion, and in that case, you have a powder of metal, and then you have a laser that basically melts it to make a layer, and then a wiper blade comes over and puts more powder on top, and then it just keeps melting successive layers. So additive manufacturing does have a lot fewer constraints as far as what your design can look like. It can have internal structures, and it can even be hollow inside, as long as you can get the powder out. But the way that generative design makes parts now tends to not make hollow parts, so a lot of them can be CNC machined.
Host: What are some of the challenges and limitations of the evolved structures process?
McClelland: It’s evolving really quickly. So basically every CAD package out there is pursuing generative design in some respect, and the evolved structures process right now is mostly tailored towards a piece of software called Autodesk Fusion 360, and the limitations of that are slowly falling away as technology matures, but right now they are, it’s really only good for isotropic materials, so things like metals and plastic. It’s not good for things like composites that have different properties in different directions, though there are pieces of software that are developing to be able to use that. It’s also not good for making tubes. So a hollow tube is a great structure often, and you tend not to get those with the current generative design algorithms.
And also, they don’t — the tools that I’m using, at least — don’t consider thermal in the equation, so you usually have to take it to one limitation or another. So a lot of times, our structures, either they want them to be thermally isolating, so not have a lot of heat transfer across them, in which case, this is usually pretty good because you get minimum-size members because it’s lightweight, and if you make it out of titanium, then it’s a pretty good isolator. Or on the other hand, you want it to have a certain amount of heat transfer, in which case you just have to basically specify, ‘I need this much of a heat path’ but it doesn’t take into account — thermal simulation is not part of the simulation currently.
Host: What’s the plan going forward?
McClelland: So, the plan going forward is to — at least on the structures front — is to try to whittle away at all of the barriers to adoption, and also whittle away at the limitations. So one of the limitations that I didn’t mention is it doesn’t do multi-part structures right now. So if you think of a truss made of composite tubes and metallic nodes, it’s not going to come up with that by design.
So, one of the things that I’m looking at for, for instance, like the next generation large space telescopes, is how to use generative design to quickly come up with the kind of structures that they might use, and there’s a couple ways that you could do that. You could come up with a structure that’s monolithic and then sort of manually slice it into different parts, or you could potentially look at ways of fabricating really large space structures monolithically. So if you look at the work of a company called Relativity Space, they’re printing an entire rocket, 3D-printing an entire rocket, which is pretty amazing. So maybe you can build these structures monolithically, or maybe you divide them up. So also looking at things like flexures and optics, and really just expanding the range of applications to which evolved structures can be applied.
Host: In terms of a timetable and what you anticipate, do you think we’re starting to already see a paradigm shift in the way that people are doing engineering design as a result of these technologies?
McClelland: Well, it’s really interesting to see how this will be adopted in industry broadly versus aerospace. So if you’re a car manufacturer, or you design bicycles, you don’t design a lot of new parts for bicycles, those are pretty well defined already, and you may design one or two. But with what we do, especially at Goddard, all our instruments are unique. Every time we design an instrument, there are dozens of these structures, and I imagine across NASA, there are just, at any time, there are thousands of these kind of structures being worked on that are unique, because almost all of our cost is non-recurring engineering, right? So there’s only one Hubble, there’s only one Webb. So I think these technologies are really uniquely suited to the work that we do at NASA, and I think that it’s so powerful.
Really the interest that I’ve been getting across the agency with this technology has really taken me by surprise, and I do expect that it’s going to start to change things, because it’s just that 10X reduction in time and that 3X improvement in performance, you don’t usually see those kind of gains in mechanical engineering. So yeah, I think it’s going to start to have an impact.
Host: This has been incredibly fascinating, Ryan. Thank you so much for taking time to talk with us today.
McClelland: Sure, it’s been great talking to you.
Host: You have any closing thoughts?
McClelland: Sure, just if folks are interested in this, they can just do some Googling on generative design, and reach out to me directly if you have applications or want to learn more, want to take a look at the specifics of the evolved structures process, I’ve written a guide that sort of walks new folks through how to leverage it.
Host: Ryan’s bio, links to topics discussed during our conversation, and a show transcript are available at APPEL.NASA.gov/podcast.
If you like the podcast, please follow us on your favorite podcast app and share the episode with your friends and colleagues.
As always, thanks for listening to Small Steps, Giant Leaps.