January 29, 2010 Vol. 3, Issue 1
The SPIRE instrument on the Herschel Space Observatory is providing a 12 billion-year glimpse back in time.
The Herschel Space Observatory (HSO) team had a vision to explore the earliest stages of star formation. Today, the Spectral and Photometric Imaging REceiver (SPIRE), one of three HSO cameras that began making observations in November 2009, is facilitating these visions with its ability to see the submillimeter sky. (“Submillimeter” refers to a portion of the electromagnetic spectrum that spans microwaves and far-infrared.) The capability of this instrument has set the floor for future space-based instruments of this kind.
Half of the Picture
In the mid-1990s, the COsmic Background Explorer (COBE) released data and images supporting theoretical models of the Big Bang. However, these images only told half of the universes story.
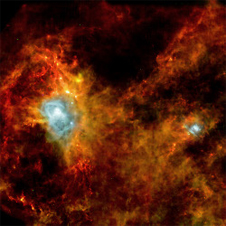
A dark cloud inside the Aquila constellation over 1000 light-years away. The image is a product of SPIRE and the Photodetector Array Camera and Spectrometer (PACS) on board Herschel. Image courtesy of the European Space Agency.
The COBE data revealed large amounts of extragalactic background light coming from the far-infrared and submillimeter part of the spectrum — so much, in fact, that it accounted for half of the light represented in the picture. (The other half consists of optical and ultraviolet light.) COBE’s instruments didn’t have the resolution to determine the source of the light. “If that light was coming from galaxies,” said Jason Glenn, co-investigator on SPIRE at the University of Colorado at Boulder, “we were missing half of the picture.”
The detectors capable of viewing submillimeter light are called bolometers. COBE had fewer than ten on board. “If you want to understand how galaxies form and evolve,” added Glenn, “you had better be able to account for that light.”
Observing the submillimeter sky is difficult for three reasons: atmosphere, temperature, and technology. The Earth’s atmosphere gets in the way of ground-based measurements, thermal emissions from the telescopes themselves add noise, and the instrument technology is challenging. Bolometer detector technology was in its infant stages when COBE was under development. It wasn’t until the detector technology started to dramatically improve in the late 1990s that astronomers had a chance at filling in the other half of the picture COBE started.
Enter SPIRE.
A Cut above the Rest For Now
SPIRE was designed to better explore star and galaxy formation by looking at emissions from clouds and dust. Since it switched on last year, this instrument, a collaborative effort led by Cardiff University and the Rutherford Appleton Lab in the United Kingdom, with major contributions from the Jet Propulsion Lab (the U.S. lead) and the University of Colorado at Boulder, has had an outstanding performance record resulting from the teams sound design, engineering, and teamwork.
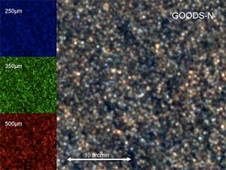
A false-color image of thousands of distant, extremely luminous, unresolved galaxies as seen by SPIRE. Color coding information indicates the temperatures and distances of the galaxies. Image courtesy of the European Space Agency and the HerMES consortium.
The SPIRE team promised to deliver an instrument that held to very tight mass, volume, and power requirements. “We had little or no margin on anything,” said Jamie Bock, the U.S. Lead Investigator on SPIRE at JPL. “Right after we were selected was the period of greatest technical stress on our team because then we had to deliver what we promised.”
While the space-based nature of the mission enabled the team to worry less about the problems posed by atmosphere and heat emitted by the telescope, the bolometer detector array remained a concern. With a growing explosion in the number of detectors possible in an array, the team would have to make a decision about the size of their array and stick to it.
SPIRE has three arrays totaling 337 detectors — more detectors than any other instrument of its kind in space, at least for now. “It’s not a lot if you compare that to buying a one million pixel CCD (Charged Coupled Device) camera off the shelf at an electronics store,” remarked Glenn, ” but it’s a huge leap forward for us because the numbers of bolometers flown on satellites before us numbered in the few.” (Glenn’s Ph.D. thesis project had only one detector.)
Today, the number of bolometer detectors one can put into an array is increasing dramatically. “Far-infrared detector technology is developing rapidly,” explained Bock, “faster than Moore’s law for computer chips.” Today’s ground-based telescopes are capable of housing larger arrays consisting of 2,000 to 10,000 detectors. Space-based telescopes are behind the ground-based telescope curve. “You get one shot when you go into space,” said Glenn, “so you have to choose detector technology that is currently state-of-the-art, but won’t be in five years.”
Testing
The SPIRE team had its share of challenges: fabricating the bolometer array and a repairing a broken Kevlar suspension were just a few. The clich “the hard stuff is easy and the easy stuff is hard” rang true, but didnt confine the SPIRE team’s ability to persevere. Solving the problems that arose depended largely on individual workmanship, where mistakes may be mundane, but lethal. It worked for SPIRE. “We had simply superb people at every stage, and especially at the critical assembly steps,” said Bock.
John Mather and Dennis McCarthy talk about the challenge of closing the requirements and the importance of testing on the COBE mission. See the full Masters with Masters event here. Video Credit: NASA
Additionally, said Glenn, being able to test everything was a big challenge. The SPIRE team was faced with the usual time constraints that simulating the space environment poses, but SPIRE’s array of detectors were also relatively new to space exploration.
Unlike optical instruments containing CCDs, little is known about the effects of space on bolometer detector arrays. “We already know how CCDs work,” said Glenn, “They’re extremely well understood. You can build them and pretty much count on them working the way you anticipate, whereas in our case the detector technology was just barely ready when we started to design and build the instrument.”
This meant that the team had to perform additional tests that evaluated detector lifetime and radiation hardness. Even though the team had a tight testing schedule that limited thoroughness, “We were certainly confident that the instrument performance was going to be good when we had to go,” said Glenn.
It turned out to be better than good.
A Global Endeavor
The early success of SPIRE on HSO is due to the successful collaboration of more than eighteen institutes from eight countries. The team faced the communication challenges posed by email, telecons, and ITAR regulations. Bock attributes the team’s ability to navigate these restrictions to the solid personal and technical connections with their English and French colleagues.
“Everyone’s goal on the project was to produce the best science within the constraints of the space instrument — that was the common culture, more than anything having to do with national boundaries,” said Bock.
In addition, said Glenn, the technical and managerial leadership for SPIRE was strong, hard working, and organized. The most difficult aspect of the overseas collaboration was frequent travel and supporting the instrument testing remotely. Echoing Bock, Glenn added, “Everyone’s primary goal was to get good performance from the instrument to enable the astrophysics we wanted to do with it.”
Said Bock, “My own takeaway is that projects can succeed with a high level of technical risk if these risks are well understood, carefully managed, and there is good communication.”