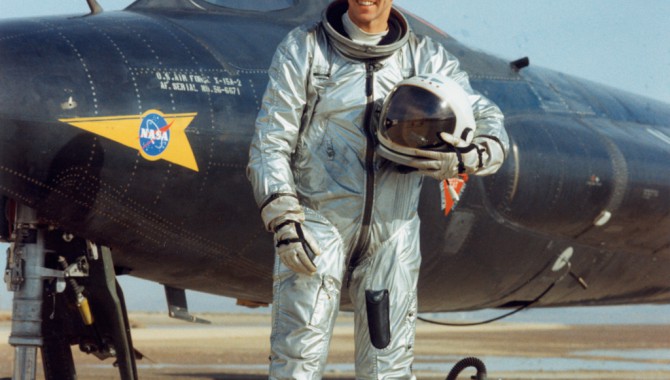
By Kerry Ellis
Breaking the sound barrier took guts, curiosity, optimism, and some serious risk taking.
Equipped with slide rules and other fifties technology, the army, navy, and North American Aviation teamed up with the National Advisory Committee for Aeronautics (NACA) to create an aircraft that could outfly all others. Through a series of experimental aircraft, NACA—later NASA—pursued supersonic and hypersonic flight with record-breaking results. The lessons learned during this ambitious research program contributed to many other NASA programs.
During 199 flights between 1959 and 1968, the X-15 achieved many firsts and set a slew of world records, some of which still stand. Those achievements required a high level of risk and included failures along the way. But the program’s ability to build, test, fly, and repeat in quick succession made every new plane better than the one before. With the freedom to rapidly churn through data and rebuild when necessary, the team achieved more than anyone imagined possible.
Building a Rocket Plane
The X-15 was created to explore hypersonic (generally defined as five times the speed of sound) aerodynamic performance, research structural behavior during high temperatures and pressure, study stability and control during exit from and reentry of the atmosphere, and examine pilot performance and physiology. What the program discovered directly contributed to Mercury, Gemini, Apollo, and the Space Shuttle.
Its immediate predecessor, the X-2, had been designed to achieve Mach 3. Pilot Milburn “Mel” Apt managed to push the plane to Mach 3.196 before turning back to Edwards Air Force Base to land; the roll he initiated to make the turn caused an adverse yaw problem previously experienced by Chuck Yeager in the X-1. The plane tumbled out of control, and Mel died in the accident. Making the leap from the tragic final flight of the X-2 to a plane intended to double the X-2’s design speed was risky. But the tragedy made the X-15 team work hard to ensure pilot safety.
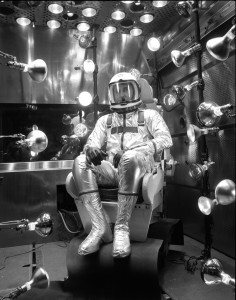
Scott Crossfield sits in a thermal-vacuum chamber during tests of a prototype XMC-2 pressure suit. Production versions of this suit were used for thirty-six early X-15 flights.
Photo Credit: Boeing
To solve the yaw problem, the team developed a wedge-shaped tail: narrow at the front and wide at the back. This helped keep the air streams apart longer at hypersonic speeds, which gave the plane exceptional directional stability. The wedge tail is now a commonly accepted shape for hypersonic control surfaces, but the X-15 was the first to employ it on a manned aircraft.
To barrel through the air at six times the speed of sound and reenter the atmosphere from the edge of space, the X-15 body needed to survive extreme stress and heat. Engineers used Inconel-X, a heat-resistant nickel-chrome alloy, and titanium for the structure—achieving the first use of a reusable superalloy structure capable of withstanding hypersonic reentry. In the process, the team developed new fabrication techniques for machining, forming, welding, and heat-treating Inconel-X and titanium.
Heating at hypersonic speeds presented other design challenges as well. Since metal expands at high temperatures, slots were built into the sides of the plane to accommodate a few inches of expansion. “It expanded just like an oilcan,” pilot Maj. Gen. Joe Engle said. “It sounded like someone banging on the side of a plane with a sledgehammer, and the old guys wouldn’t warn you about it.”
Early inertial systems affected how the X-15 was eventually flown. The systems relied on gyros, whose axes would be thrown off kilter during hypersonic acceleration. This effect prevented pilots from knowing how high or how fast they were flying. To accurately measure thrust energy, the team decided to time how long the engine burned. A stopwatch in the cockpit told pilots when to shut off the engines in order to complete a planned flight path with precision. Burning the engine for one second too long could mean an extra 280 mph and 35,000 ft. of altitude, something pilot Maj. Gen. Bob White experienced firsthand.
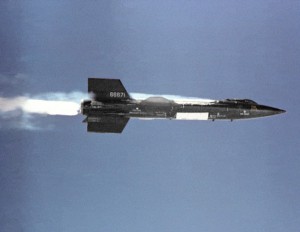
Above: The X-15 research aircraft during its first powered flight on Sept. 17, 1959.
Photo Credit: NASA
Piloting Through Near Space
Engle described the X-15 team as one that worked together in perfect harmony: “If I didn’t look at badges, I would not know who anybody worked for on that program; it was that kind of operation.” The pilots who undertook the flight risks were key members of that team.
To keep pilots safe from the immense heat and pressure experienced during flight, engineers developed nitrogen cabin air-conditioning for the cockpit. This also helped counteract potential flammability should the engine malfunction. While filling the cockpit with oxygen was an option, the potential for an explosion if it interacted with the engine’s flammable propellant was too high a risk. To prevent blackouts from high g-forces and ensure the pilots could breathe within the nitrogen-filled environment, the X-15 team developed an early pressure suit, also pressurized and cooled with nitrogen. The only breathable oxygen available was in the suit’s faceplate.
Each flight lasted about eight to ten minutes. Around eighty seconds after the X-15 was launched from under the wing of a B-52, pilots would shut down the engines. The rest of the flight was unpowered, and the pilot was essentially guiding a highspeed glider. Precision piloting was critical. If the engine was cut one second too late, or if the pitch was off by one degree, pilots could end up thousands of miles off track. With the only navigation available being line of sight from the X-15’s two small windows, a few ground stations, and non-hypersonic chase planes, overshooting the course meant emergency landing decisions had to be made and communicated quickly. And if one of the windows glazed or cracked from the heat of flight—a problem that often occurred—pilots were reduced to tracking on only one side of the plane. This made the stopwatch in the cockpit very important for accurate energy management and flight-path positioning.
Eight pilots were given the title of astronaut for flying more than 50 miles above the earth’s surface. One, Captain Joe Walker, set an aircraft altitude record of 67 miles above Earth. When they were flying in near space, “Airplane attitude didn’t make much difference,” Engle said. “You could fly sideways or backwards over the top. But it was very important once you started back down and got into sensible atmosphere to be lined up both in pitch and yaw for reentry.” At the wrong angle, the plane could skip up during reentry, which happened to pilot Neil Armstrong during one flight. “He ended up getting down to Burbank, turning around, and barely made it in to the dry lake bed, landing south to north instead of north to south like usual,” described Engle. “It was the longest flight in duration we had in the X-15.”
Training was key. Laying a foundation for later space programs, the X-15 program relied on a ground simulator to help pilots grow familiar with the minute controls and precise timing required for hypersonic flight.
A HEART-POUNDING EXPERIENCE
A major concern when NASA began to explore human spaceflight further was the physiological responses of those chosen to fly the missions. Heart rates for pilots were very high, and data from X-15 pilots directly influenced the decision to fly men into space. Below is Walter C. Williams’ account of how the heart-rate issue was finally resolved.
We were working hard on Project Mercury. We were getting ready to fire Alan Shepard on the first ballistic flight. Prior to that, we had a little hearing before the President’s Scientific Advisory Committee. It had two types of members: engineering types and aeromedical types.
We had a terrible time with the doctors; that’s the only way to describe it. They thought we ought to fly seventy-five more chimps before we flew a man. I’m serious! We had the data from this one chimpanzee, which showed very high pulse rates, and they were concerned that this might kill a man or you’d pass out or what have you. And so we had quite a go-around on that.
Meanwhile, the X-15 was flying out here and the pilots were being monitored and, yes indeed, they had high pulse rates due to stress; their highest rates were usually before launch or landing. So I sent out for that data and brought it in and for a while I thought they were going to cancel the X-15 instead of clearing us to fly Project Mercury!
So Don Flickinger, the senior research aeromedical doctor, and one who had been closely following the X-15 program, got one of the doctors on the committee and Joe Walker in a three-way conversation (the data we had involved Joe Walker). The doctor began questioning Joe about this and that, then saying, “These pulse rates are pretty high—over 150. How did you feel?”
Joe responded, “Oh, I felt all right. Now wait a gosh-damn minute. Are you trying to ask me whether or not I fainted?”
The doctor said, “Well, yes. Did you faint?”
Joe replied, “Hell, no! I didn’t faint!”
The doctor continued, “Well, I don’t know people can pass out and not realize it.”
Joe retorted, “Look, what I did one second depended on what I had done the second before, and I’m here talking to you!”
Paving the Way for Human Spaceflight
The pilots and engineers on X-15 worked together to discover the magic altitude where flying with reaction controls—rocket thrusters on the nose and wings—would work best. “Engineers asked us to find out where that magic altitude was,” said Engle. “We made some errors coming back in—sometimes getting a bit of roll. We learned that a blended, adapted flight-control system, using both reaction controls and aerodynamic surfaces, is necessary for an entry from space back into the atmosphere. The same flight-control system developed on X-15 was used on Space Shuttle.”
The way the Space Shuttle lands was directly influenced by the X-15’s unpowered landings. “Early in the design phase of shuttle, there was a very strong feeling that we really shouldn’t be landing something with as low a lift-to-drag ratio as the Space Shuttle unpowered,” explained Engle. “In fact, one of the early design proposals had air-breathing engines that would fit into the aft end of the payload bay. They would have taken up a quarter to a third of the payload bay in volume, not to say how much it was going to weigh, for the engines to be on a mechanism to fold out after you went supersonic, start up the engines, then fly it in like a conventional airliner. That plus the fuel.” Being able to demonstrate safe, accurate, unpowered landings with the X-15 proved to shuttle developers that a conventional powered landing was unnecessary.
Pressure suits developed for the X-15 provided insight into how full-pressure suits restricted the reach and energy envelope—how much and with what effort suited pilots could move—which paid off later in the design of spacesuits. “There was considerable discussion with Wright Field concerning use of a partial-pressure suit, which was developed, versus a full pressure suit, which had to be developed,” said Walter C. Williams, chairman of the X-15 Flight Test Steering Committee. “It was felt important to develop a full-pressure suit. This suit became the foundation on which suit technology was built for use in the space programs.”
The X-15 program also demonstrated the first application of hypersonic theory and wind-tunnel testing to an actual flight vehicle, which helped provide confidence in the wind-tunnel studies later done for the shuttle.
Pushing the Envelope
The X-15 program, and its team, achieved so much in so little time due in large part to their skill and willingness in taking measured risks, pushing the envelope of what could be done with high-speed flight. The results of their collaboration paved the way for human deep-space exploration, influencing Mercury, Gemini, Apollo, and the Space Shuttle. Risk-taking was vital for the team’s leaps in innovation.
“There is a very fine line between stopping progress and being reckless,” said Harrison Storms, chief engineer at North American Aviation during the X-15 program. “The necessary ingredient in solving a sticky problem is attitude and approach. [It’s] what I refer to as ‘thoughtful courage.’ If you don’t have that, you will very easily fall into the habit of fearful safety and end up with a very long and tedious-type solution at the hands of some committee. This can very well end up giving a test program a disease commonly referred to as ‘cancelitis,’ which results in little or no progress and only creates another ‘Hangar Queen.'”
More Articles by Kerry Ellis
- Engineers Without Borders (ASK 39)
- NextGen: Preparing for More Crowded Skies (ASK 38)
- Science from the Sky (ASK 36)
- Innovating to Fly in Cleaner Skies (ASK 35)
- SOFIA: Getting Airborne (ASK 33)
- + View More Articles