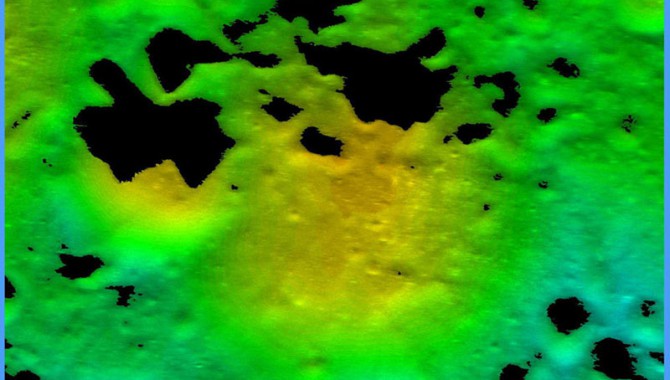
By Haley Stephenson and Matthew Kohut
When NASA announced that the Lunar Reconnaissance Orbiter (LRO) would upgrade from a Delta II to a larger Atlas V launch vehicle, a window of opportunity opened for an additional mission to the moon. The Atlas V offered more capacity than LRO needed, creating space for a secondary payload.
The Exploration Systems Mission Directorate (ESMD) posed a challenge to interested secondary-payload teams: the chosen mission could not interfere with LRO; it could not exceed a mass of 1,000 kg; it could not cost more than $79 million; and it had to be ready to fly on LRO’s schedule. Of the nineteen proposals submitted, ESMD chose the Lunar CRater Observation and Sensing Satellite (LCROSS)—a mission that sought to search for water on the moon by firing a rocket into the lunar surface and studying the debris resulting from the impact.
LCROSS was not about pushing the technical envelope. It was about keeping it simple—keeping it good enough.
Ames Research Center served as the lead center for LCROSS. Dan Andrews, LCROSS project manager, was charged with assembling a team that could develop a satellite on a shoestring while coordinating its efforts closely with LRO. “It could have been a real recipe for disaster,” he said. “There were plenty of reasons why this mission should not have succeeded.”
The Good-Enough Spacecraft
From Andrews’s perspective, the LCROSS spacecraft had to be “faster, good enough, cheaper.” He made clear to his team from the beginning that LCROSS was not about maximum performance. “It was about cost containment,” Andrews said. “LCROSS was not about pushing the technical envelope. It was about keeping it simple—keeping it good enough.”
The LCROSS team had twenty-nine months and $79 million to build a Class-D mission spacecraft. Class-D missions, which are permitted medium or significant risk of not achieving mission success, must have low to medium national significance, low to medium complexity, low cost, and a mission lifetime of less than two years. The low-cost, high-risk-tolerance nature of the project led to a design based on heritage hardware, parts from LRO, and commercial off-the-shelf components.
LCROSS’s status as a Class-D mission did not preclude the team from practicing risk management. “We were risk tolerant, but that doesn’t mean we were risk ignorant,” said Jay Jenkins, LCROSS program executive at NASA Headquarters.
“With the LCROSS instrument testing, we shook, cooked, and cooled the mostly commercial off-the-shelf parts that could potentially come loose during launch so that we were likely to have a tough little spacecraft, but we didn’t test to failure,” said Andrews.
LCROSS consisted of a Shepherding Spacecraft (SSC) and a Centaur upper-stage rocket. The SSC included a fuel tank surrounded by a repurposed Evolved Expendable Launch Vehicle (EELV) Secondary Payload Adaptor, also known as an ESPA ring. The ESPA ring, conceived by the Air Force Research Laboratory as a small-satellite deployment system, had never been flown on a NASA mission. Its six bays can hold up to six small satellites, but on LCROSS those bays held the principal subsystems of the spacecraft. Using the ESPA ring offered a significant advantage: its sturdy design had already been tested, facilitating flexible, low-risk integration with the LRO mission.
The off-the-shelf payload instruments included imaging equipment found on army tanks, carpet-fiber recycling hardware, and instruments used to measure engine-block temperatures for NASCAR. The total instrument cost ran about 3 percent of mission cost—far lower than possible with custom-built instruments.
Andrews described this as a capabilities-driven approach. “The whole principle of LCROSS was, ‘If there are investments that the agency or industry have made and it’s something we can employ, then do it,'” he said.
While LCROSS was a Class-D mission, LRO, a Class-B/C mission, and the Atlas V rocket, a Class-A vehicle, were held to much higher standards. The launch vehicle provider expressed concern that LCROSS might interfere with the performance of LRO, the primary payload. “It became a lowest-common-denominator problem,” said Andrews.
LCROSS didn’t have the budget to meet the requirements of a Class-A mission, nor was it supposed to. Andrews raised the issue with the program office, which eventually facilitated some additional testing of its structural hardware to ensure that LCROSS satisfied the concerns of all the mission stakeholders.
With good lines of communication, the two teams started to view each other as resources and “worked together like a team this agency hasn’t seen in a long time.”
Teamwork
Andrews knew he had to establish trust with the other teams involved in the mission. LRO, based at Goddard Space Flight Center, was understandably cautious about LCROSS hitching a ride to the moon with them. Andrews quickly moved to identify an LCROSS engineer who could take up residence with the LRO team to facilitate quick dialogue and build trust between the two missions. With good lines of communication, the two teams started to view each other as resources and “worked together like a team this agency hasn’t seen in a long time,” said Andrews. “These good relationships really pay off when things get tough.”
The crossover and reuse of hardware between the LCROSS and LRO spacecraft allowed the teams to learn from and with one another. Sometimes they worked in tandem; at other times one team would be ahead of the other. “There were things that we missed that we either caught later, or missed and LRO caught, and vice versa,” said LCROSS Project Systems Engineer Bob Barber.
A good partnership with Northrop Grumman (NG), the spacecraft contractor, was also essential. Neither Andrews nor NG Project Manager Steve Carman had ever managed spacecraft development before, though both had run spaceflight-hardware development projects. “We were both kind of new to the spacecraft side of things, but I told my management to provide me with an outstanding team, and Dan did the same,” said Carman.
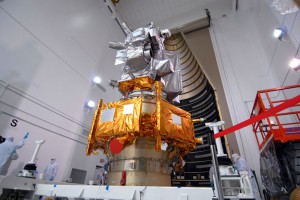
At Astrotech Space Operations Facility in Titusville, Fla., LRO and LCROSS are united in preparation for fairing installation. Photo Credit: NASA/Jack Pfaller
During the first six months, as the project underwent some acquisition-related contractual changes, Andrews and Carman began to develop a mutual trust. “Ultimately, communication was the hallmark of the partnership,” said Carman. “The partnership was not something where we said, ‘Sign here—we are partners.’ It grew out of a relationship. … We showed them as we went along that we were indeed capable of doing this faster than anything we had done here.”
For Andrews, trust grew out of a shared understanding of the way both organizations traditionally operated. “We talked plainly about budgets. We talked plainly about the NASA construct, and then we talked plainly about how hard it is to move NG’s heavy institution,” he said. “I was not holding anything back in terms of what I was sharing with them, and I think that set a tone within NG [so] that they behaved similarly.”
By the time of the preliminary design review, a cooperative dynamic had been established that went beyond business as usual. “It was an ‘open kimono’type relationship where everything was kind of on the table,” said Barber. “We wanted a really open and honest relationship with them.” NASA team members took part in NG’s risk management boards and were invited to staff meetings.
The relationships didn’t end when people left the project. Both NASA and NG experienced turnover, which could have hurt the project. In this case, though, several former team members kept in touch with their successors. “That’s when you know a team is more than just coming to work and doing stuff,” said Barber.
Tightening the Schedule
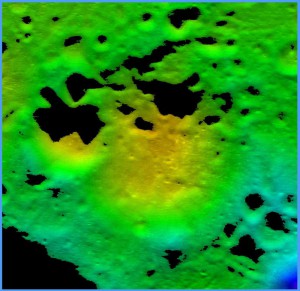
Close-up image of crater Cabeus A near the moon’s south pole showing crater elevation. Yellow represents lower elevations.
Photo Credit: NASA/Jet Propulsion Laboratory
To meet the aggressive schedule demands of LCROSS, Carman established a baseline project plan with very little margin and then challenged key team members to consolidate their subsystem schedules. “We had a schedule that was based on ‘When do you need it?’ and I was saying, ‘How fast can you do it?’ And so people found ways to modify the processes,” said Carman.
For example, the lead propulsion engineer came back to Carman and said she could pull six weeks out of the propulsion schedule. As the work progressed, the team continued to make gains, eventually ending up eight weeks ahead.
Expediting the Review Process
The LCROSS schedule didn’t allow time for a lengthy review process throughout the project life cycle. Andrews and Carman orchestrated a compromise that reduced the number of NG internal reviews and made the review process more collaborative.
Prior to each key milestone review, the teams held a peer review, which they called a design audit. Since both NASA and NG wanted to send managers and experts to check on the project, Pete Klupar, head of the Independent Review Board for NASA, jokingly threatened to give a short quiz at the beginning of the reviews to determine which stakeholders had done their pre-meeting reading and study. This made the point that the purpose of the reviews was not to educate the stakeholders, but to draw on their expertise.
By inviting stakeholders to the critical design audit near the end of Phase C, the team experienced a relatively smooth and quick critical design review. This process was so successful that the team then applied the same concept to the validation and verification process by instituting verification compliance audits. “This very informal, hands-on, roll-up-the-sleeves, no-ties-allowed stakeholder involvement right from the get-go is all reflective of that collaborative process,” said Jenkins.
Risk Tolerance in Practice
The LCROSS team had to determine how far it was willing to go with risks. Too many changes to the spacecraft could turn an acceptable risk into one that was too great.
Early in the project, the team discovered that a capacitor responsible for protecting voltage input to a field-programmable gate array (FPGA) was identical to one that failed in the power system. If the capacitor regulating voltage to the FPGA failed, the FPGA would experience voltage stress; it was unclear how much stress the FPGA could handle. Loss of this FPGA would be a fundamental, unrecoverable problem, potentially ending the mission altogether.
“All the probability analysis said this should be a very low risk,” said Barber, “but it was a mission killer if the wrong one failed.”
The capacitor was built into a box that had passed all its testing and was performing well. The problem was that the location of the capacitor made remote viewing of its condition impossible. With little room for error in the budget or schedule, the team didn’t want to invite more risk by opening up a tested flight box to check the capacitor, which could very well be fine. This was one of the most challenging risk trades this project would have to navigate. It wasn’t until a change in the Atlas V launch manifest led to a delay in the launch date that the LCROSS team had the time and resources available to revisit its risk list. The team determined that the risk of going in to test the capacitor was lower than doing nothing at all.
“We took a risk [opening the box] to try and eliminate what we felt was our highest risk [the capacitor]. Then we ended up closing that risk, and we took it off the plate,” said Barber.
Against long odds, the project met its cost and schedule constraints and passed its final reviews. It was time for launch.
Low on Fuel
The Atlas V launched LCROSS to the moon on Tuesday, June 18, 2009. One hour after launch, LRO separated from the rocket to head toward the moon and insert itself into lunar orbit. LCROSS took another path.
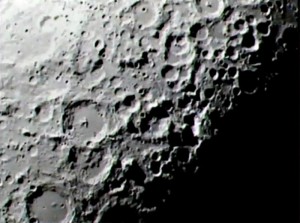
The LCROSS mission operations team initiated power-up of the LCROSS science payload and saw this view of the moon.
Photo Credit: NASA
Two months into its journey to the moon, LCROSS experienced an anomaly while the spacecraft was out of contact with NASA’s Deep Space Network. The spacecraft’s inertial reference unit—its onboard gyro and primary means of measuring rotation rates around each axis for attitude control experienced—a data fault that resulted in the spacecraft’s thrusters firing propellant almost continuously.
The operations team noticed this once the spacecraft was back in contact with the network. Engineers quickly identified a probable root cause and contributing factors. Immediate steps were taken to stop the thrusters from firing and prevent a similar occurrence. The team also adopted new ultra-low fuel consumption means to conserve propellant. The specific cause of the fault remained unresolved, but the engineering teams determined that the spacecraft would have enough propellant to achieve full mission success even under worst-case conditions.
Smashing Success
Six weeks later, LCROSS lined up on its collision course with the moon. Once in position, the Centaur rocket separated from the SSC and barreled down toward the moon’s Cabeus crater, where it crashed at twice the speed of a bullet. Following minutes behind the Centaur, flying through the vapor cloud created by the LCROSS impactor, the SSC took pictures, analyzed the debris, and sent the data back to Earth before it, too, smashed into what turned out to be a soft, porous crater floor. The whole sequence, lasting a mere four minutes and nineteen seconds, went off without a hitch.
More Articles by Haley Stephenson
- The Next Big Thing Is Small (ASK 38)
- Gettysburg Addressed: Common Ground for NASA Engineers and Civil War Generals (ASK 36)
More Articles by Matthew Kohut
- Sharing Knowledge About Knowledge (ASK 38
- Rocketing from the Past to the Future (ASK 37
- Building the Team: The Ares I-X Upper-Stage Simulator (ASK 34)
- A New Design Approach: Modular Spacecraft (ASK 33)
- The Quest for Good Ideas (ASK 33)
- + View More Articles